

SEQUENCE CONTROL + RECOGNITION
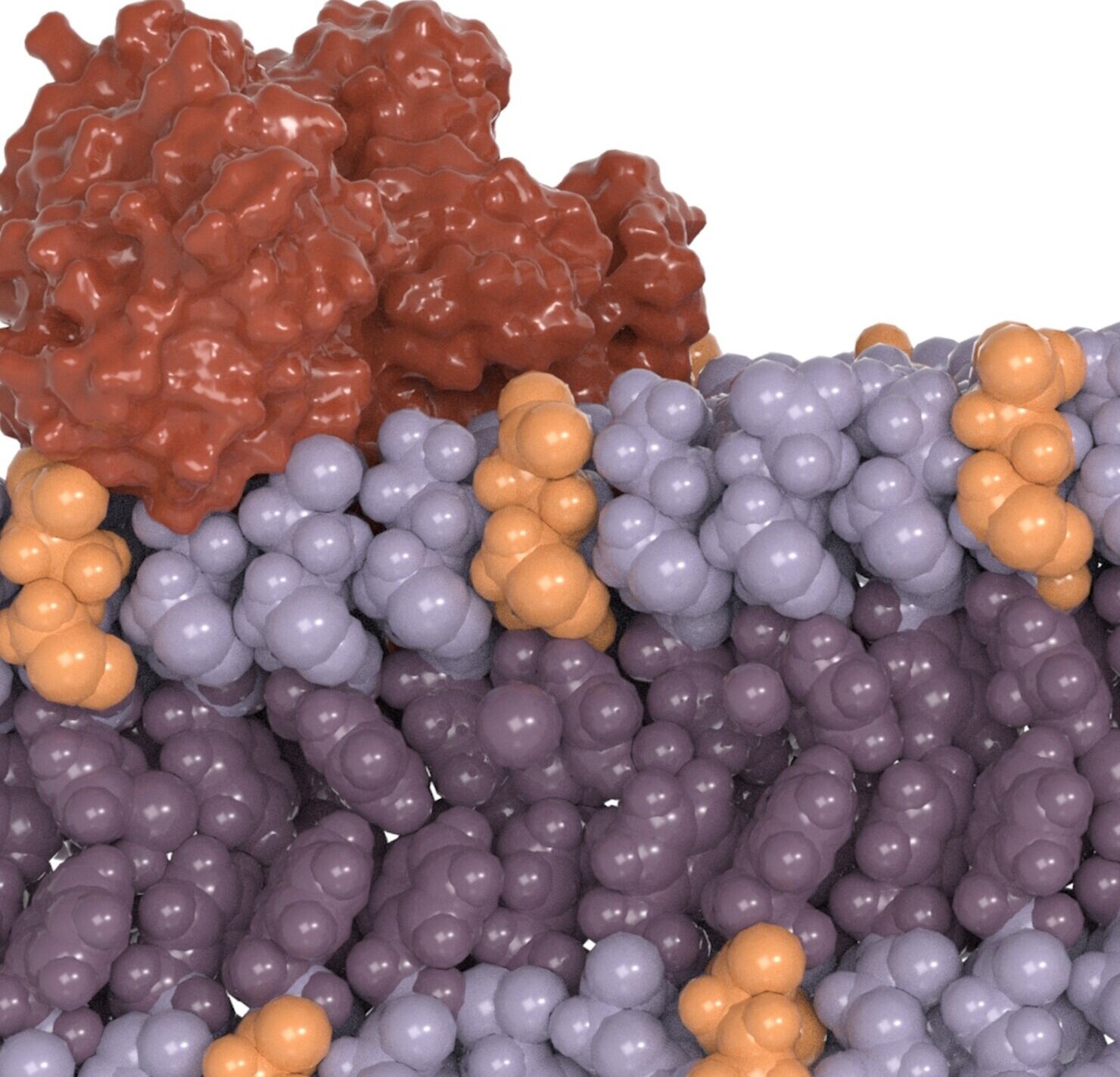
SOFT + COMPATIBLE

BIODEGRADABLE + RECYCLABLE
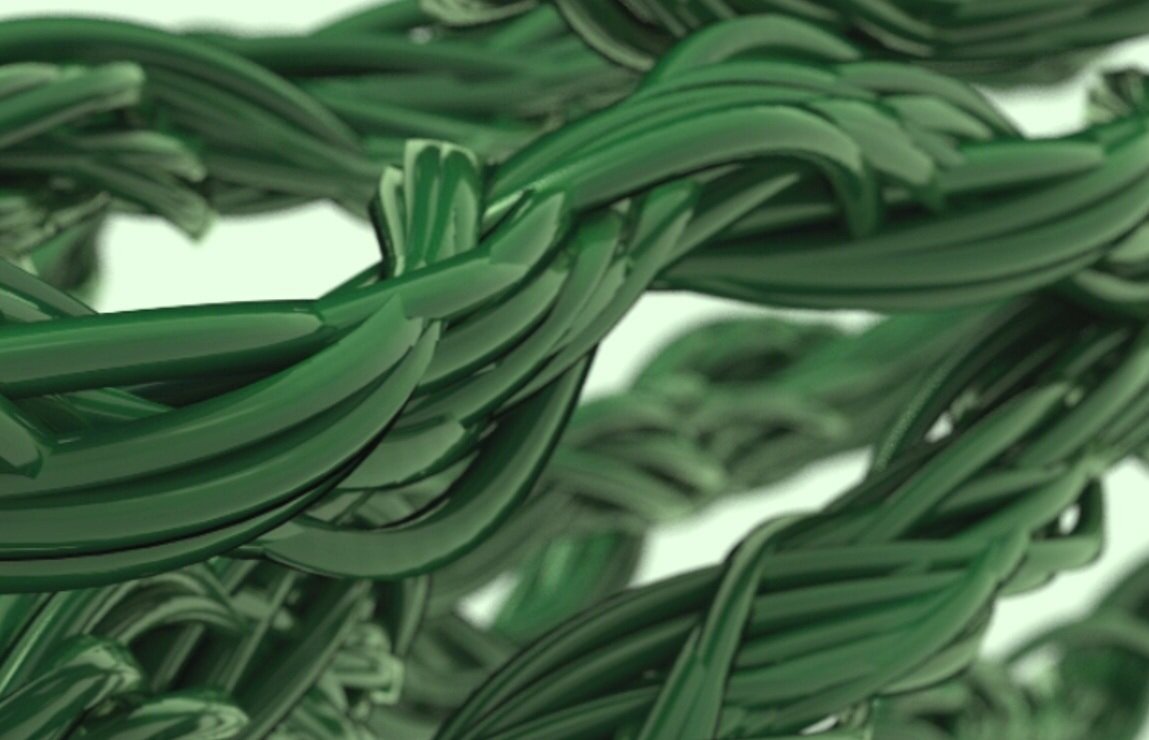
AUTOMATED + ACCELERATED

POLYMER CHEMISTS <> MOLECULAR ARCHITECTS
OUR INSTRUMENTATIONS
I am pretty happy with most of my equipment, as it is a balance between price and availability. One of my favorite instruments is the automated recycling GPC— something I did not have in my PhD and postdoc. It is awesome! I also think the Vigor glove boxes are just as good (so far!), and much more competitive price wise. I also quite like the Gyros PurePep for our solid-phase synthesis. A bit expensive but maybe we will get more reactors in the future for the space. It is particularly good for programming of peptoids. I really avoided getting a UV-Vis but it was essential for our time studies, so we thank Agilent for the discount through the award. We got a Teledyne ISCO flash, which I normally like, but I got a demo unit that is sub-par (weaker pump, cannot do very large columns; lacking a setting so the nozzle leaks upon disconnecting). In retrospect, I wonder if we should’ve stuck with the classic Biotage for microwave reactions. I liked the automation feature in CEM, but we noticed the seal is not as robust and had a hardware failure within two years (and no way we can fix it ourselves). For a lab with high throughput, the automation may be useful.
Happy to chat with anyone who wants more details on these instruments!
to be purchased/moved soon:
2-port glove box
6 port-glove box
High temperature GPC
Room temperature GPC
Keithley 4200 and probe station